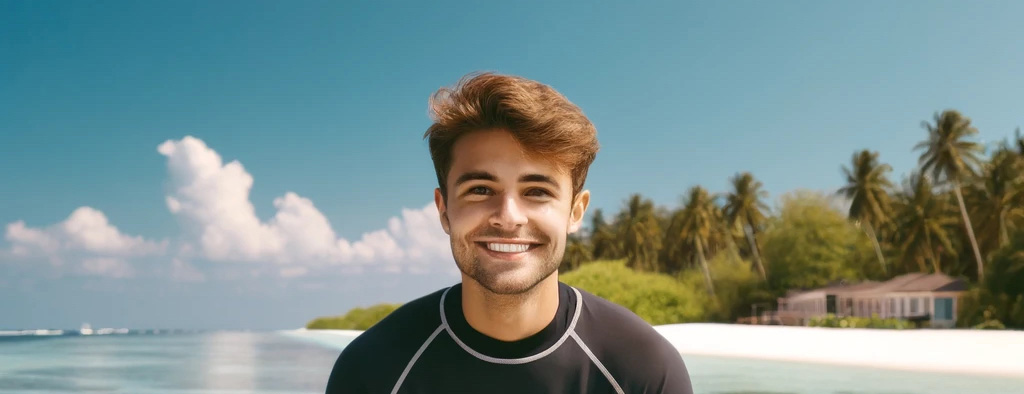
Armed with a basic understanding of the respiratory and circulatory systems, gas exchange, and how they function on the surface, the next step is to examine gas exchange under pressure.
In your SNSI Open Water Diver course, you were introduced to the concept of breathing gases under pressure. This section of the SNSI Advanced Open Water Diver program will review those concepts, and take a deeper look into what they mean for divers.
William Henry (1775-1836), an English chemist, who studied, among other things, gas solubility, found that, at a constant temperature, the amount of gas that dissolves into a fluid is directly proportional to the partial pressure of the gas. This is called Henry’s Law.
As previously discussed, gases transfer into the blood due to the pressure gradient between the alveolar wall and the pulmonary capillaries that surround the alveoli. When a diver is submerged under water, the regulator supplies gas at ambient pressure. As such, the pressure inside of the diver’s alveoli is approximately the same as the surrounding pressure. This means that the gas pressure inside of the lungs will increase in proportion to the depth of the diver. Consequently, the pressure gradient between the gas in the alveoli and the gas in the pulmonary capillaries is higher, which means that more gas will enter solution in the bloodstream, and more gas will be transferred to the tissues.
Henry’s Law goes on to state that the speed at which the gas dissolves into a liquid depends on the affinity between the gas and liquid. This is a vital factor for divers because the human body is composed of many different tissues and mediums. Each of these manages oxygen and nitrogen loading and unloading differently in response to ambient pressure changes. Henry’s Law affects all of the gases contained in the breathing gas; however, the management of nitrogen is the most important factor for the recreational diver. For this reason, most of the focus will be about nitrogen and how it behaves in a divers body.
A gas will diffuse into a fluid or tissue until it has reached a state of equilibrium. When this state is reached, the tissue has become saturated. The speed at which a tissue will become saturated by a specific gas, in this case nitrogen, is referred to as the saturation half time. Nitrogen’s saturation half time is the time it takes for a specific tissue to become half saturated with nitrogen. The value of the tissue’s nitrogen half time is determined by the affinity that each specific tissue has for nitrogen, and is independent of the pressure.
For example, a hypothetical tissue, called tissue x, has a nitrogen half time of 20 minutes. In this example, it will take 20 minutes for tissue x to become half saturated at 10 meters / 33 feet. At 20 meters / 66 feet, it will take the same amount of time, 20 minutes for tissue x to become half saturated. This is because half time is in reference to volume, not the amount of gas contained within the tissues due to pressure. Using the above example, at 10 meters / 33 feet, after the first half time has passed, the tissue will be half saturated with exactly double the amount nitrogen molecules than there would be on the surface. At 20 meters / 66 feet following the first half time, the tissue would be half saturated with triple the amount of molecules than on the surface, etc. Complete tissue saturation occurs over an exponential growth curve. In practice, tissues become half saturated in the first half time, and by half of a half in the second half time, and so on. It takes approximately seven complete half times for a tissue to become completely saturated.
Remember that each type of tissue has its own half time for nitrogen. The time it takes for muscle tissue to on board nitrogen is not the same amount of time it takes adipose (fat) tissue, they each have a specific half time.