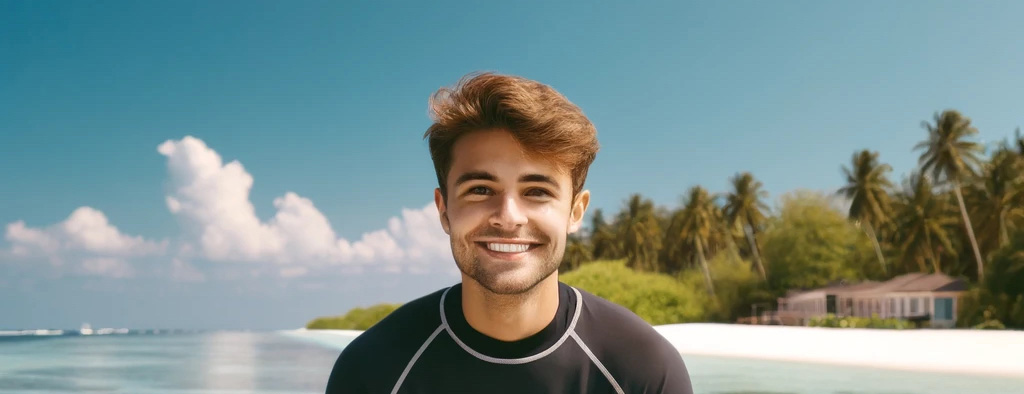
The function of the Respiratory System is to transfer oxygen from the air we breathe into our blood and eventually to our cells.
Our cells require a regular supply of oxygen in order to function properly, and in turn, they create carbon dioxide as a byproduct that must be removed and expelled from the body.
To know how respiration works as a system, we must first have a basic understanding of the parts involved and what they are responsible for.
The Airways
The Respiratory System is made up of airways, alveoli, arteries, capillaries, veins and neuromuscular controls that keep it all regulated and functioning correctly.
The Airways include the nose, mouth, larynx, trachea, main right and left bronchi, bronchi, bronchioles and terminal bronchioles. This all ends up in microscopic sacs called the Alveoli where gas exchange takes place. Like grapes on a vine, the alveoli are attached to the bronchioles.
The alveoli are millions of tiny balloon-like sacs, each made up of a very thin membrane wrapped in a dense net of capillaries.
The alveoli, and their associated capillaries form the foundation of the pulmonary system, as they are the primary point of gas exchange. Combined, the total alveoli in an average adult would cover approximately 83 square yards / 70 square meters.
The Neuromuscular System
The Neuromuscular System is a generic term that encompasses the respiratory center of the brain and the respiratory nerves and muscles.
The ribs protect the lungs, among other organs, and are designed to work with the respiratory muscles to help the lungs expand and contract. The primary respiratory muscles are the dome shaped diaphragm, and the intercostals.
The diaphragm is located at the base of the rib cage and separates the thoracic cavity from the abdominal cavity. The intercostals are the muscles that lie between the ribs. Some neck and chest muscles assist with breathing; however, they are considered secondary.
The respiration center of the brain, located in the medulla oblongata and pons, is the central control center that regulates all aspects of breathing.
The primary stimulus that dictates our breathing rate and depth is the amount of carbon dioxide (via acidity) detected in arterial blood.
When the level of carbon dioxide is high, the respiratory center sends an increasing number of signals to the respiratory muscles, stimulating them to increase frequency and depth of respiration until the detected level of carbon dioxide has decreased.
Once the levels have stabilized, respiration returns to normal levels. Emotional factors, such as anxiety, panic and stress can cause increases and decreases in respiration rate, as can chemical and pharmacological mechanisms.
Inhalation is an active process; it requires us to spend energy by contracting our muscles. During inhalation, the intercostal muscles contract, which cause the ribs to expand. The diaphragm also contracts, flattening and lowering it closer to the abdominal cavity. The lungs respond to the increase in space by expanding, which lowers the internal lung pressure, as compared to the atmosphere. This pressure differential causes air to move into the lungs.
Conversely, exhalation is a passive process. Once the respiratory muscles relax, the ribs retreat and the diaphragm returns to its normal shape and location, increasing the pressure inside the lungs, allowing the air to passively escape.
The Gas Exchange
As previously noted, the air we breathe under normal conditions is composed of approximately 79.02 percent nitrogen, 20.94 percent oxygen and one percent trace gases, such as argon, carbon dioxide and water vapor. The more approximate value of Nitrogen, under normal circumstances is 78.08%. Carbon Dioxide is 0.04%. The remainder is trace gases.
The respective partial pressures, expressed in mmHg are:
– Oxygen = 159,144 mmHg,
– Nitrogen = 600,552 mmHg,
– Carbon Dioxide = 0.304 mmHg.
As expected, the percentage, and therefore, the partial pressure of oxygen and carbon dioxide are notably different in exhaled air. The approximate percentage of oxygen in exhaled gas is 16,3%, and carbon dioxide is 4,5%. This equates to partial pressures of:
– Oxygen = 123.88 mmHg,
– Carbon Dioxide= 34.2 mmHg.
There is little to no change between inhaled and exhaled nitrogen, as the body does not use it for any purpose. However, nitrogen is absorbed, and is present in the blood and tissues of the body, which if unchecked, could potentially cause problems for divers. In fact, the additional nitrogen that is absorbed by our tissues when we dive is the primary reason we have to follow specific depth and time guidelines to avoid decompression sickness.
Gas exchange takes place between the capillaries and alveolar wall through the respiratory membrane. The exchange is facilitated by a pressure gradient — the difference in the partial pressure of oxygen in the alveolar gas and the partial pressure of oxygen in the blood. The same is true for the exchange of carbon dioxide.
Gas tends to travel across permeable membranes where a gradient exists, from a higher pressure to a lower pressure, until equilibrium is achieved.
Blood traveling from the heart via the pulmonary artery has a lower concentration of oxygen and a higher concentration of carbon dioxide compared to the concentrations of freshly inhaled air in the lungs. At the respiratory membrane, oxygen moves from the alveolus, across the respiratory membrane, and into the blood. Carbon dioxide moves from the capillary, across the respiratory membrane, and into the alveolus, to be exhaled. The process for gases to reach equilibrium takes about 0.25 second in a normal individual. The same type of process takes place on the cellular level, and is called systemic gas exchange.
When the oxygenated blood reaches the capillaries of tissue beds, the oxygen moves from the arterial blood, due to the higher concentration of oxygen in the blood, through the cellular walls, to the cells that have a lower concentration of oxygen.
The cells produce carbon dioxide as a byproduct of metabolism, which is expelled from the cell body into the capillary due to the carbon dioxide gradient.
After this process, the blood is deoxygenated venous blood and travels back to the heart, where it is pumped to the lungs via the pulmonary artery and the process starts over.
The Carbon Dioxide
The Pulmonary Arteries carry blood with a smaller partial pressure of oxygen, and a higher partial pressure of carbon dioxide from the heart to the capillaries in the lungs, where under normal circumstances, the carbon dioxide is expelled and the oxygen is replenished.
The Pulmonary Veins then take the oxygen rich blood from the lungs, back to the heart to be distributed to the rest of the body by way of arteries.
Red blood cells, which are contained within the blood plasma (fluid), carry the oxygen molecules. These cells contain a substance called Hemoglobin, which has the ability to chemically bind with many different gas molecules; oxygen is among its favorites. When oxygen passes through the respiratory membrane, it binds to the hemoglobin almost immediately.
Under normal circumstances, gases do not exist as bubbles in the blood. Gas transports within the blood is conducted on a molecular level. Oxygen, nitrogen and carbon dioxide exist in the blood as molecules, and in some cases they bind to other molecules.
Carbon Dioxide can be extremely dangerous if its partial pressure increases beyond a certain threshold. Therefore, it must be expelled from the body quickly. As we’ve discussed, the amount of carbon dioxide in the blood is the primary factor that dictates respiratory rate under normal circumstances.
Hypercapnia, the excess of carbon dioxide in the blood, can occur for many reasons including difficulty breathing, and short, shallow breathing. In the case of a diver, a poorly maintained or malfunctioning regulator may cause carbon dioxide levels to rise.
Short, shallow breathing causes a buildup of carbon dioxide faster, because the majority of gas that is typically inhaled and exhaled during a short, shallow breath is the gas in the anatomic dead space; the space between the mouth and the bronchioles that does not reach the alveolar gas exchange.
If this shallow breathing is prolonged, the exhaled air, which has a higher content of carbon dioxide, remains in the anatomic dead space, and is returned to the alveoli with each successive inhalation, increasing the severity of the hypercapnia. A diver who skip-breathes; takes a breath less often than he should in an effort to save gas, will also cause the carbon dioxide levels to significantly rise in his body.
The increased density of the inhaled air, and the increased resistance of exhalation due to the increased density of gas while diving may also cause poor gas exchange. For example, imagine a room with one door that is half full of people. It is easier and faster to clear out than a room full of people, because if the room is full, there are twice as many people to clear out.
The gas a diver inhales becomes denser as the he dives deeper. Denser gas is harder to inhale, and it takes more effort to exhale. Poorly maintained equipment can exacerbate difficulty inhaling and exhaling denser gas.
The symptoms of hypercapnia often include headache, nausea and drowsiness. More severe cases may include tremors, chest cramps and unconsciousness.
The immediate treatment for hypercapnia is the administration of 100% oxygen, by a qualified oxygen administrator. Emergency medical services should be contacted any time a diver’s level of consciousness diminishes, or he is, or becomes unconscious.
To help avoid hypercapnia, it is recommended to use high quality, well-maintained equipment and breathe normally — deeply and slowly, without interruption for the duration of the dive. Avoid diving deeper than the dive plan calls for, and avoid excessive multiple deep dives in a single day.
Anxiety, stress and panic while underwater can lead to hyperventilation — breathing deeply and rapidly, resulting in the reduction of carbon dioxide in the body. Hyperventilation can be voluntary, or in the case of panic, involuntary.
Lowering the level of carbon dioxide in the blood and tissues results in a depressed stimulus to breathe. Free divers often hyperventilate before a dive so that they can hold their breath longer without the physiological impulse to breathe.
Hyperventilation, whether intentional, or unintentional can be very dangerous. Prolonging apnea — a temporary discontinuation of breathing, after excessive hyperventilation, slows down the stimulus to breathe. This may result in hypocapnia — reduced carbon dioxide in the blood, and loss of consciousness. Unintentional hyperventilation as a result of anxiety or panic can quickly get out of control and lead to greater hyperventilation and eventual unconsciousness.
The best way to prevent hypocapnia is to breathe normally for the entire dive. If you find yourself breathing deeper and faster than normal, stop, think, relax and breathe normally.
The Circulatory System
The Circulatory System is responsible for transporting blood, nutrients and waste throughout the body. It is composed of the heart, arteries, veins and capillaries.
The heart is a hollow muscle that under normal circumstances, rhythmically contracts to push blood through the arteries to all areas of the body. The blood returns to the heart through the veins, which contain one-way valves to prevent backflow of blood, enabling the heart to basically draw the blood back.
The heart is the primary organ of the circulatory system and is located in the thoracic cavity between the lungs and just above the diaphragm. The heart contains four cavities: the right and left atria, and the left and right ventricles.
The atria are oriented above the ventricles, and are the smallest of the four chambers. The heart is divided longitudinally, in that the right atrium and right ventricle are separate from the left atrium and left ventricle.
Between the atria and ventricles are one-way valves called atrioventricular valves, or AV valves. Blood enters the heart atria from the body and lungs by way of veins. Blood leaves the heart ventricles and is pumped to the body and lungs. Because of this, blood in the arteries is considered to have a centrifugal — moving away from center, motion, while blood in the veins is considered to have a centripetal — moving toward center, motion.
As arteries get father away from the heart, they become arterioles. Arterioles branch out abundantly from the arteries and are much smaller in diameter. Form there, the arterioles continue to wane in diameter and become arterial capillaries.
The capillaries are so small that red blood cells have to line up one after another to get through them. As the red blood cells pass through, they deoxygenate and the capillaries become venous capillaries. The capillaries are the junction point of the arteriole and venous systems. The smaller vessels then merge into larger diameter vessels called veins.
Typically, arterial blood is considered to be blood that is oxygen rich, and venous blood is considered to be oxygen poor. There are two notable exceptions to this, the pulmonary artery and the pulmonary vein.
The pulmonary artery originates from the right ventricle of the heart and carries blood high in carbon dioxide to the lungs. Inside of the lungs, the pulmonary artery branches off into numerous vessels that eventually form a web like network of microscopic arterioles around the walls of the alveoli.
The blood is then oxygenated as the red blood cells pass through the capillaries and returns to the heart via the pulmonary vein. The pulmonary vein empties into the left atrium of the heart.
The blood is pumped by the heart muscle through the left AV valve (bicuspid valve), into the left ventricle, which then pumps the oxygenated blood into the aorta to be distributed to the rest of the body via arteries and arterioles.
In the larger principle arteries, blood travels at approximately 30 centimeters (1 foot) per second, due in large part to the elastic properties of arteries. Once the blood reaches the arterioles, the speed slows to less than a millimeter per second to allow for gas exchange to occur. At this point, gas, nutrient and waste transfer takes place between the tissue and blood.
The blood moves through the veins and returns to the heart’s right atrium through the vena cava. It is then passed to the right ventricle were it is sent to the lungs to be re-oxygenated via the pulmonary artery.
While this covers what we need to know as divers about inspiration and expiration, the actual physics and physiology is much more deeper.